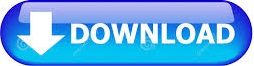

“The assumptions in those models concerning the physical environment can therefore be refined, which will eventually lead to a better understanding of the physics of supernovae.” “Our analysis shows that some models that investigated the production of high-energy neutrinos in supernovae from a theoretical perspective predicted too many of these particles,” adds Necker. With newer survey instruments and next-generation neutrino telescopes planned for the future, the presented limits will only improve and may even lead to a detection. In order to improve sensitivity, they also performed a stacking analysis that looked for a combined excess from the catalog as opposed to looking at individual sources.Īlthough no significant correlation was found between neutrinos and the SN catalog, the researchers were able to set strong upper limits on the contribution of SNe to the neutrino flux. The researchers also included a parameter that described the number of detected neutrinos from the direction of each SN. To do this, the researchers compared the hypotheses where 1) there is high-energy emission from SN and 2) there is no signal, therefore the neutrinos must come from sources other than the supernovae included in the study. “Because most of the neutrinos in IceCube are not produced in space but come from interactions of high-energy particles in the Earth’s atmosphere, we have to be careful to not pick up a correlation that just happens by chance,” said physics PhD student Jannis Necker at the Deutsches Elektronen-Synchrotron and one of the lead analyzers of the study. They then correlated the catalog with 700,000 events recorded by IceCube. Credit: IceCube Collaborationįor the analysis, collaborators first compiled a catalog of supernovae from publicly available records, totalling more than 1,000 SNe. Because the lines are below the gray band we can say that the contribution of supernovae to the astrophysical neutrinos must be subdominant. The yellow, red, and blue lines show our upper limit on the contribution that can come from different subtypes of supernovae. The gray band shows the astrophysical neutrinos that IceCube measured. The figure shows the spectrum of high energy neutrinos, the flux of energy per time, area and solid angle. No evidence for high-energy neutrino emission was found, but stringent upper limits were set on the SN contribution to high-energy neutrino emission. In a new study submitted to The Astrophysical Journal Letters, the IceCube Collaboration presents a search using different SN classes as potential neutrino sources. Now, IceCube is looking for similar neutrino emission at much higher energies. The first detection of neutrino emission from a supernova (SN) came from low-energy neutrino detectors that observed Supernova 1987A 35 years ago, the brightest extragalactic supernova in history. Although the majority of extragalactic neutrinos detected by IceCube have come from outside our galaxy, the question of more precisely where still remains. From these interactions, high-energy neutrinos can be produced, which are the subject of study for the IceCube Neutrino Observatory, a gigaton-scale neutrino detector at the South Pole. The ejecta from the CCSN can then interact with the dense circumstellar medium where charged particles can be accelerated. Called core-collapse supernovae (CCSNe), these explosions are triggered by the implosion of the dying star’s core. When a star reaches the end of its thermonuclear evolution, it explodes, giving rise to neutron stars and black holes.
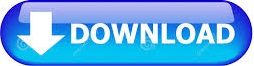